Learning what can degrade heat-transfer-fluid quality can help minimize potentially negative effects
Organic heat transfer fluids (HTFs) are robust workhorses that are quite stable both thermally and chemically. However, they can yield to attacks from powerful or sometimes even subtle forces, falling short of the hopes and expectations of system owners. The best defense against these attacks is to know the strengths of a fluid and how to strategically intervene. This guide will help educate system owners on how to successfully resist these attacks and minimize impacts of those which may be already underway.
Basic guidelines
Maintaining the fluid quality will minimize its change from like-new condition. In a new system, the first goal is to have the system free of potentially problem-causing debris and residue prior to filling the system with the new heat transfer fluid. It is common for piping and equipment to have a thin rust-inhibitor coating on the metal surfaces that guards them from rusting prior to commissioning. Practical experience has found that small amounts of these coatings have a relatively insignificant impact on the fluid chemistry and stability. As a result, there should be no need for chemical or detergent cleaning and rinsing of the equipment. Of course, any gross contaminants or problem residues, such as from a repurposed vessel, should be locally cleaned.
Preparations before filling. Prior to filling a new system, it should be leak tested — typically with water — to ensure system tightness before filling with the HTF. When draining the water, it is expected that there will be a small amount left behind. Efforts should be made to minimize the residual water and to remove it via warm gas purging or similar methods to greatly facilitate startup. Some use an exit dew point of –30°F (–34°C) as an indicator that the gas-drying step is complete. Excessive water content in the HTF will require a boiling-out process to force the moisture to flash into the vapor phase and be vented from the expansion tank vent. Depending on the system design, this could be a lengthy process. However, it is an essential task to support operation of the fluid at temperatures well above the boiling point of water. Water itself will not react with organic heat transfer fluids within their intended operating temperature ranges. Drying of the excess water from a system leaves the heat transfer fluid with low parts-per-million concentrations of water and undamaged from the experience. Alternatives to hydrostatic testing with water include pressurized gas with soap solution and low-pressure helium leak detection.
Preventing oxygen exposure. With the fluid heated to temperatures above 175°F (80°C), an organic fluid should be protected against exposure to oxygen in air. This can be accomplished by providing an inert gas blanket within a closed expansion tank or ensuring the fluid temperature within the expansion tank remains cool enough to resist oxidation. The proper design for inerting is to establish a static blanket of positive internal pressure. This keeps oxygen out of the system and protects the fluid from oxidation [ 1]. If a continual purge or sweep of gas is provided, this can gradually deplete the heat transfer fluid of its lower-boiling components, shift the average molecular weight of the fluid higher, and lead to increasing viscosity. The fluid composition and performance can change for the worse, deviating from like-new condition — sometimes to the point that a partial replacement of fluid will be needed to adequately restore its performance. The change from new to compromised fluid composition from this mechanism is illustrated in Figure 1.
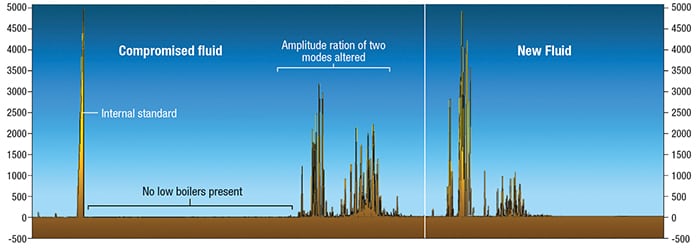
Figure 1. On the right, the gas chromatogram of a new heat-transfer fluid with bimodal distribution of peaks is displayed. The left shows fluid after inert gas purging has partially depleted the lower-boiling components (peaks have been shifted to left for illustration). Note that there are no low boilers present to the far left, indicating essentially a complete void of low-boiling degradation products due to the continual gas purge
A secondary route of vulnerability to oxidation is when heated fluid is drained from the system. Even if collected in clean and dry vessels, oxidized fluid should not be returned into the system. A best practice to permit continued use of drained fluid is to establish job plans that allow the fluid to be cooled to less than 175°F (80°C) prior to draining into clean vessels. Cooling to below 140°F (60°C) will further protect personnel from potential thermal burns in case of skin contact.
Thermal stresses. Next, the potential for excessive thermal stressing of the fluid should be considered. The first consideration of fluid selection is to properly match the fluid to the requisite temperature requirements. A fluid operating above its thermal stability limitation will be damaged by the routine requirements of the process. A properly rated fluid should have the ability to continuously operate at the required supply temperature to the process heat users while providing long and acceptable life [ 2]. In the real world, circumstances sometimes create unplanned high-temperature excursions beyond the maximum temperature rating of the fluid. If the maximum bulk-temperature rating of the fluid exceeds the heat-transfer-fluid supply temperature requirements, the fluid has built-in capacity to withstand some short-duration and limited temperature spikes or excursions. Causes of these temperature spikes can include heater-firing controls overshoot, flow imbalance within the heater coils, and power failures that interrupt fluid flow through the heater. Should these circumstances exist, efforts should be made to identify their causes and implement corrective measures before the accumulating impacts of these events irreparably damage the fluid. Some solid-fuel-fired heaters may be more vulnerable to temperature spikes due to the nature of the fuel; hence, a fluid with excess thermal stability capacity is expected to be more resistant to degradation.
Typically, low-temperature extremes should not damage an organic heat transfer fluid. Fluids composed of multiple components will not transition into a crystalline solid form, but will experience increasingly higher viscosity as the temperature decreases. Some fluids with a small number of components, such as eutectic biphenyl and diphenyl ether blend, will freeze into a solid form. On warming, the organic fluid chemistries return undamaged to their liquid state. Organic fluids that do not freeze into crystalline solids should also pose no risk of freeze damage to piping or tubing, which is common with water ice.
Contamination. A potentially more immediate chemistry change of a fluid can result from in-leakage from a process-side stream of greater pressure. This ingress is immediately apparent if the process stream is aqueous and at temperatures above 100°C, since the water can rapidly vaporize and expand to hundreds of times its liquid-phase volume. Smaller leak rates of aqueous streams can be diagnosed by the onset of pump cavitation, increased system pressures, and audible gurgling sounds inside the piping and expansion tank. While the water itself should not chemically interact with the heat transfer fluid, the other process stream components may create issues, such as insoluble solids or flash-point depression, or by introducing thermally unstable organics that quickly break down and either foul heat transfer surfaces or deposit coke within heater coils. Consideration should be given to potential system impacts if such process contamination occurs, with heat-transfer-fluid selection criteria evaluating the ability to best manage the contamination with the least negative consequences. Figure 2 illustrates a mixture of relatively immiscible fluids of differing densities.
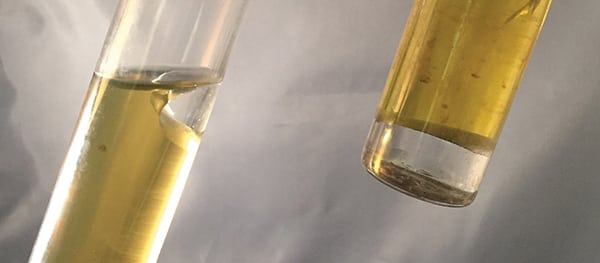
Figure 2. Fluids of differing density and solubility can result in localized issues based on position of the potentially troublesome layer
Mixing fluids. Just as process contamination can be accidental, the accidental addition of the wrong make-up fluid can happen as well. Actual examples of this unplanned mixing include the addition of drums of glue and JP-8 jet fuel into a heat-transfer-fluid system. Typically, other organic chemistries are of lower thermal stability and can break down quickly. If they break down into lower-boiling compounds, it may be possible to vent to a safe location from the system expansion tank. Higher-boiling compounds formed will often contribute to sludge formation. Some compounds can rapidly form coke deposits in the highest temperature zone of the fluid within the heater coils. If this occurs, the cooling action of the heat transfer fluid is greatly reduced and can cause the coil wall temperature to increase, leading to blistering or rupture. Consequences can be rapid and severe, so action in response to process contamination and improper make-up fluid addition should be prompt.
Another example of improper fluid addition into a heat-transfer-fluid system is the return of condensate collected from the system vent stream. While this condensate was once within the system itself, it may have chemically changed due to oxidation within the vent condensate collection tank. Return of the fluid into the system will introduce organic acids into the system. These acids can increase the risk of corrosion to carbon-steel components and increase the viscosity of the fluid, which can reduce its heat transfer efficiency. Of course, if water was present in the returned condensate stream, the evolution of water vapor within the system will be immediately apparent. If the condensate collection tank is shared among multiple processes, the stream can also contain contaminants from other processes.
Keys to Maintaining Heat-Transfer-Fluid Quality
- Match heat transfer fluid to the process temperature demands.
- Avoid problem-causing contaminants.
- Protect against heat-transfer-fluid oxidation and excessive purging and venting.
- Identify and correct causes of high-temperature excursions.
- Establish a working relationship with heat-transfer-fluid supplier technical resources.
- Establish and maintain appropriate administrative and engineering controls.
Consequences
What are the consequences of heat-transfer-fluid quality changes? With increases of acidity from either process contaminants or fluid oxidation, the carbon-steel components of the system are at increased risk of corrosion. Most commonly, this is first observed in the vapor space region of the expansion tank where topside nozzle welds can develop pinholes. If the expansion tank is open to the atmosphere and its contents are below 100°C, some organic fluids with lower density than water will allow the water and acids to collect in the bottom of the tank, leading to vessel bottom corrosion (see Figure 3). As previously noted, organic contaminant degradation products, as well as those of significantly degraded heat transfer fluid, can lead to the precipitation of insoluble solids and the formation of deposits onto heat exchange surfaces. These conditions can increase fouling resistance to heat transfer and reduce the thermal efficiency of the system [ 3]. In severe instances, the solids can obstruct instrument sensing lines and increase risk of premature pump-seal failures if solids lodge in-between seal faces. Many times, oxidized fluid experiences a significant viscosity increase, reducing its heat transfer efficiency and increasing energy usage. If the heater-outlet-temperature set point is adjusted upward to compensate for the reduced efficiency, increases to fuel usage, costs, and the thermal degradation rate of the heat-transfer-fluid result.
Some foreign contaminants can have such low thermal stability that they quickly form coke deposits within heater coils, leading to coil overheating, blistering and possible rupture. Monitoring of heater performance to detect the onset of such consequences can include heat-transfer-fluid temperature rise, pressure drop, and flowrates through each coil pass of the heater. With properly balanced flowrates, these measured parameters should be common to each pass. Monitoring of heater stack-gas temperatures, fuel usage rates, and coil skin temperatures using infrared imaging can also be helpful.
Some HTF chemistries can exhibit increased thermal degradation rates when affected by elevated concentrations of impurities or contaminants that can act as free radicals to promote molecular changes [ 4]. As a result, the usable life of the heat transfer fluid is diminished. When production units cannot afford to shut down for a proper identification and repair of leaking equipment and replacement of the fluid, some have chosen to perform partial fluid replacements online. This is a costly approach to extend the operation until a planned downtime, and the subsequent total fluid replacement remains necessary. Contaminated fluid may have additional disposal issues to manage as well, such as one user whose process inadvertently partially halogenated the heat transfer fluid due to in-leakage. The named impacts to operations all act toward increased frequency of unplanned downtimes and increased maintenance costs.
Corrective actions
What if the heat transfer fluid has already fallen victim to one or more of the problems mentioned? The priority is to correct the root cause(s) that led to the deteriorated fluid quality, whether it be equipment failure, human error or other issues. The fluid quality should be assessed. This typically involves the fluid experts consulting with the heating system owner’s experts to determine if the fluid quality requires improvement. Analysis of a representative sample of the suspect fluid should be conducted. Fluid quality adjustments can involve a partial to full fluid replacement. This will dilute any contaminants, degradation products, insoluble solids, moisture, and acidity proportional to the fraction of fluid replaced. Added attention to fluid filtration can be an appropriate measure for further insoluble solids removal.
Severe situations where fouling and deposits of solids have occurred can require emptying the system for a more thorough cleaning of the equipment and piping. Options can include solvent cleaning, organic fluid flushing, or water-based chemical cleaning [ 5]. Consultation with the heat-transfer-fluid supplier can be helpful in determining the approach best suited for restoring the system cleanliness, while minimizing costs of cleaning and fluid disposal and keeping the turnaround time to a minimum.
If the heater coils may have been compromised, one useful technique is thermal scanning. While limited to designs permitting the view of an imaging camera through an inspection port for visibility of coils near the combustion zone, this approach can be used to trend coil skin temperatures over time to assess the potential ongoing accumulation of internal coke fouling. The coke can act as an insulation layer, resisting heat transfer into the fluid. As a result, the coil wall or skin temperature increases compared to normal. With diligence in periodically measuring coil skin temperatures, it is possible to have early warning that can prompt corrective measures prior to coil failure. It is important to realize that without baseline temperature measurements when coils are free of coke, measurements taken later with coke deposits present cannot be judged as “normal” or “elevated.”
If the heat transfer fluid is being excessively thermally stressed due to process temperature requirements being beyond the capability of the fluid to withstand, alternate fluid chemistries should be considered. Consultation with the heat-transfer-fluid supplier can usually identify at least one or two viable alternative fluids of greater thermal stability to consider. With any upgrade of fluid chemistries, a variety of engineering evaluations and actions should be undertaken, including potential changes in fluid volume expansion (expansion tank sizing), materials of compatibility, pressure-relief-device sizing, heater design calculations, pump sizing, instrumentation calibrations, hazard communication training for personnel, and more.
In the follow-up to any event that compromised the heat transfer fluid, corrective actions should be implemented to prevent recurrence. These can be in the form of administrative controls, such as refresher training, revised procedures, new process signage, or updated policies focused on intervening in the identified causes. They may also include engineering controls, such as modified control system logic, equipment changes, operating condition changes, or changes of the heat transfer fluid itself.
Final thoughts
Perhaps the best approach to managing fluid quality is prevention of the common problem causes. This starts with a good system design that can manage the effective venting of moisture and low-boiling thermal degradation products from the system. It also requires knowledge of the optimal expansion-tank design features. Any proposed heat-transfer-fluid system design or modification should be reviewed by an expert for comments and insights on the ability of the design to support fluid maintenance.
Next, it is important to establish a good fluid sample-analysis monitoring program, beginning with a baseline sample in the first month of operation. Samples should always be collected from the same sample port for consistency and from a location where the fluid is well mixed. Ask the fluid supplier for the recommended sample frequency, but annual sample analysis is most common for routine monitoring. Intermediate samples should be considered if process upsets are suspected to be fluid related.
Even a properly designed and constructed system can have problems if operated and maintained inadequately. Ensure the personnel responsible for the operation of the system are knowledgeable and are provided with adequate training to avoid or minimize excursions that can impart stresses to the fluid and system components. When concerns are raised, they should be promptly investigated so that corrective actions can be implemented before problems become undesirably large or costly to address.
Lastly, system owners should establish a good relationship with their fluid supplier’s representatives. These experts have vested interest in supporting their products’ users to maintain their satisfaction, to optimize the performance and life expectancy of the heat transfer fluid, and to bring technical specialist resources to assist their customers quickly and effectively — usually at little to no added cost. The user of the heat transfer fluid should utilize this important expert resource, which is at their disposal, so that their focus can remain on their own production metrics.
Edited by Dorothy Lozowski
References
1. Technical Information Bulletin No. 4, Heat Transfer System Expansion Tank Design, Eastman, p. 3.
2. Liquid Phase Systems Design Guide, Eastman, p. 4.
3. Therminol® Information Bulletin No. 2, In-Use Testing Of Therminol Heat Transfer Fluids, Eastman, p. 3.
4. Gamble, C. and Schopf, M., “Optimization Opportunities for Therminol® VP-1 Heat Transfer Fluid in Concentrating Solar Power Facilities,” Solutia Inc., 2009.
5.Therminol® Information Bulletin No. 1, Cleaning Organic Heat Transfer Fluid Systems, Eastman, p. 3.
Note: Eastman brands referenced herein are trademarks of Eastman or one of its subsidiaries. The® symbol denotes registered trademark status in the U.S.; marks may also be registered internationally
Author
Conrad Gamble, P.E., is the product steward for Therminol heat transfer fluids at Solutia Inc., a subsidiary of Eastman Chemical Company (Anniston, AL; Phone: 256-231-8525; Email: [email protected]). Gamble joined Solutia Inc. (then Monsanto Co.) in 1985 as a process engineer. He served for 11 years in heat-transfer-fluid process engineering, manufacturing and engineering roles until his current assignment as senior technical specialist. Since 1999, he has supported the Therminol heat-transfer-fluid business in product development and customer technical service and provides customer educational training. A licensed professional engineer and recognized expert in the industry, Gamble has served the chemical industry for more than 30 years. He is also a member of the American Institute of Chemical Engineers. Gamble holds a B.S.Ch.E. from the University of Alabama in Tuscaloosa.